There is currently sufficient scientific evidence to assert that strength training is an effective method for preventing, treating and potentially reversing various chronic diseases. Indeed, adherence to a properly designed strength training program can significantly increase the physical and mental health of the population.
The importance is such that several world-renowned organizations (World Health Organization, Centers for Disease Control and Prevention, American Heart Association, American Association for Cardiovascular and Pulmonary Rehabilitation, American College of Sports Medicine) recommend this form of training for maintain health.
However, despite this evidence, most of exercise recommendations are still for aerobic training, and few physicians (and health professionals in general) recommend strength training. This article aims to alert for the relevance and valuable impact of strength training on health.
About 100% of our biological existence has been dominated by outdoor activity. Hunting and searching for food has been a condition of human life for millions of years1. That is, if in the past it took effort (i.e. physical activity) to find food, nowadays food comes to us without having to make any effort. Therefore, we have moved from a very active lifestyle to a highly sedentary lifestyle. With serious consequences for public health. If in the past all people had to engage in some sort of physical exertion to carry out their daily tasks, today most of them do not have those needs. The environment has changed and so have people. They are weaker, sicker, have more chronic pain and are increasingly dependent on medicines. But the message still going on in our society (and passed on in medical appointments) is “make no physical efforts and follow your normal life”. And I believe this is the worst advice people can get! Normal life? But what kind of advice is this? How can normal be good? You must be completely alienated from reality in order to make such recommendations.
Today we have more opportunities than ever to build a healthy and strong phenotype. The phenotype is the expression of our organism, and it depends largely on the choices we make every day. Two organisms can have the same genotype, the same DNA, but different phenotypes – based on their experiences and the environment. Admittedly, there are things we cannot control such as our genetic heritage, the place of the world where we were born / lived, overall luck and the general environment to some extent. But there are many things that we can control that depend solely on our priorities in life and our daily choices (examples: exercise habits, eating, sleeping, stress management, smoking, alcohol, exposure to polluted environments). And I believe that exercise in general (and strength training in particular) is the most important factor of all. It is the most potent, it’s quantifiable and acts quickly on all systems and organs of the human body.
The reality is this: the population is aging and with more chronic / noncommunicable diseases. The main noncommunicable diseases are cardiovascular diseases, cancers, chronic respiratory diseases and diabetes. These four disease groups alone account for over 80% of the 41 million deaths in the world2! According to the first report on healthy aging by the World Health Organization (WHO), the number of people over 60 is expected to double by 20503, and it is in this context that we need to urgently intervene to promote motor autonomy and improve people’s functional capacity. Traditional recommendations for walking, swimming, Pilates, and “doing low effort activities” or “no physical effort” probably need to be reconsidered and properly contextualized.
It is in this context that strength training and athletic training play a key role. All people (athletes and non-athletes) need to train their physical qualities to live with quality and independently. After age 30, adults lose 3-8% of their muscle mass per decade. Over time, the loss of lean mass contributes to a decrease in muscle strength and power, which are important predictors of balance, falls and mortality4. In the case of the elderly, it is important to note that falls are the main cause of accidental death after age 65 and hip fractures are those that most affect their independence5.
When I speak of strength I mean the basis for interacting with the environment around us, the foundation for the development of other physical qualities (mobility, power, speed, agility, muscle endurance), the ability to produce strength against external resistance (it can be the floor or any other object) through muscle contractions. This is probably the most trainable capacity we have and the one that could have the greatest impact on improving our function, independence and functional longevity. Tasks such as brisk walking, sitting and rising from a chair, climbing stairs, maintaining balance, carrying luggage, or playing with children / grandchildren are examples of activities in our daily lives that require a minimal component of various manifestations of strength (maximum strength, power and strength endurance). Therefore, both strength and muscle (quality rather than quantity) are physical function related parameters that need to be taken care of in the quest for achieving a healthy aging phenotype.
These issues are even more important when we note that as of 1st of October 2016, in the tenth revision of the International Classification of Diseases (ICD-10), sarcopenia has been classified as a disease by WHO and has its own code (M62.84). This should lead to increased availability of diagnostic tools and increased enthusiasm for the pharmaceutical industry to develop drugs to combat sarcopenia6. But in my opinion, this also represents a great opportunity for exercise professionals to be able to help fighting this disease, as strength training (properly oriented of course) will be the most potent stimulus in its prevention and treatment.
Pedro Correia
References:
- Booth FW, Roberts CK, Laye MJ. Lack of exercise is a major cause of chronic diseases. Comprehensive Physiology. 2012;2(2):1143-1211. doi:10.1002/cphy.c110025.
- GBD 2015 Risk Factors Collaborators. Global, regional, and national comparative risk assessment of 79 behavioural, environmental and occupational, and metabolic risks or clusters of risks, 1990–2015: a systematic analysis for the Global Burden of Disease Study 2015. Lancet, 2016; 388(10053):1659-1724.
- Beard JR, Officer A, de Carvalho IA, et al. The world report on ageing and health: A policy framework for healthy ageing. Lancet 2016;387:2145e2154.
- English KL, Paddon-Jones D. Protecting muscle mass and function in older adults during bed rest. Current Opinion in Clinical Nutrition and Metabolic Care. 2010;13(1):34-39. doi:10.1097/MCO.0b013e328333aa66.
- National Center for Injury Prevention and Control of the Centers for Disease Control and Prevention. Preventing Falls: A Guide to Implementing Effective Community-Based Fall Prevention Programs 2nd edition. Atlanta: 2015.
- Anker SD, Morley JE, von Haehling S. Welcome to the ICD-10 code for sarcopenia. J Cachexia Sarcopenia Muscle. 2016 Dec;7(5):512-514. Epub 2016 Oct 17. PubMed PMID: 27891296; PubMed Central PMCID: PMC5114626.
Check Part 1 HERE and Part 2 HERE
Implications and practical applications of calorie restriction and intermittent fasting
And now … can the practice of calorie restriction (CR) or fasting have therapeutic value in humans? As noted earlier (Part 1), randomized controlled trials in humans studying the effects of CR and fasting in humans are scarcer. It is not easy to find volunteers to subject themselves to the “discomfort” of eating less food.
Just an aside …
Changing something in someone’s diet can be a daunting task! In fact, and based on my experience, people are generally highly resistant to changing whatever their eating patterns are and tend to defend them tooth and nail! They elaborate the most varied rationales (and very sophisticated sometimes … like the typical “but my grandfather is 90 years old and always ate this and that”) to justify the intake of certain foods that, essentially, they are just used to or like eating. Nutrition is like a religion for some, believe me …!
Back to human studies …
The other practical reason for the lack of controlled randomized human trials on the effects of CR or fasting to study its effects on life expectancy and the incidence of “age-related diseases” is that human life expectancy is long. However, some randomized controlled trials in humans point to clear benefits of CR practice in certain populations. Listed below are some of these studies, type of intervention and significant effects observed.
• Wang et al. (2013)
- Sample: obese individuals.
- Intervention: 5 days of 30% CR (low-fat / high-carb or high-fat / low-carb) after isocaloric diet period.
- Significant results:
- CR diets decreased fasting insulin and leptin levels by increasing free fatty acid levels (indicating mobilization of fat stores);
- Insulin sensitivity did not improve significantly (perhaps due to the short 5-day period), however muscle insulin signaling (in response to insulin) increased only in the low-fat / high-carb diet subjects. Note that this effect on insulin signaling in response to the low-fat / high-carb diet (rather than the high-fat / low-carb diet) may represent only a transient adaptive response due to the higher glycemic load in the diet. The short duration of the study does not allow the conclusion of a sustained improvement in insulin regulation.
- Kitzman et al. (2016)
- Sample: elderly obese individuals (67 ± 5 years) with heart failure.
- Intervention: 20 weeks of CR (350-400kcal / day deficit) with or without exercise (1 hour walking 3 days per week).
- Significant results:
- Both CR and exercise (separately) increased aerobic capacity (indicated by increase in VO2 peak), with even greater effects if combined;
- Both CR and exercise (separately) improved body composition (fat loss) with even greater effects if combined;
- CR (but not exercise) reduced the inflammatory marker C-reactive protein (CRP) and correlated with weight loss.
- Snel et al. (2012)
- Sample: obese individuals with type 2 diabetes mellitus (T2DM) and insulin-dependent.
- Intervention: 16 weeks of CR (450kcal / day) with or without exercise (1 hour + 4 30-minute sessions on a cycle ergometer per week).
- Significant results:
- Both CR and exercise improved fasting glucose, insulin and glycosylated hemoglobin (HbA1c) levels;
- The RC + exercise group lost more fat and waist circumference compared to the CR group only;
- Both CR and exercise increased insulin receptor expression and signaling (revealed by muscle biopsy) as well as peripheral insulin sensitivity.
- Pedersen et al. (2015)
- Sample: overweight or obese, non-diabetic individuals with coronary artery disease.
- Intervention: 12 weeks of CR (800-1000kcal / day) with or without exercise (3 days / week interval aerobic sled).
- Significant results:
- Separately, CR was superior to exercise in weight loss, fat mass and waist circumference, as well as fasting blood glucose, insulin sensitivity and glucose tolerance. However, CR led to better results combined with the exercise program.
- Razny et al. (2015)
- Sample: non-diabetic obese individuals
Intervention: 3 months CR (1200-1500 kcal / day) with or without 1.8 g / day omega-3 fatty acids (in a 5: 1 DHA / EPA ratio).
- Significant results:
- CR with or without omega-3 supplementation resulted in similar decrease in body weight and fat mass;
- CR had a superior positive effect on triglyceride and insulin levels when combined with omega-3 supplementation;
- Sample: non-diabetic obese individuals
RC + omega-3 (but not only CR) improved indicators of insulin resistance (HOMA index).
- Prehn et al. (2016)
- Sample: postmenopausal obese women.
- Intervention: 12 weeks CR (<800kcal / day) followed by 4 weeks on an isocaloric diet or 16 weeks on an isocaloric diet (control group). Recommendation to increase physical activity per week.
- Significant results:
- CR (but not the isocaloric diet) resulted in better scores on memory performance tests;
CR (but not the isocaloric diet) resulted in improved glycemic control and HbA1c levels;
- CR-induced increase in cerebral gray matter density was negatively correlated with glucose levels.
- CR (but not the isocaloric diet) resulted in better scores on memory performance tests;
Recommendations and Conclusions
In fact, CR or fasting interventions do indeed appear to have clear therapeutic utility in improving health parameters related to obesity, inflammation, insulin resistance, oxidative stress, and cardiac function. It is important to note that just reducing the amount of food you eat may not be enough and perhaps not recommended. Such a simplistic and long-term intervention can result in nutritional deficits and thereby boycott putative positive health outcomes. It is therefore important to monitor and ensure adequate nutrient levels through supplementation and / or in choosing nutritionally dense foods. Also noteworthy are the positive synergistic effects that CR seems to have when combined with exercise (Snel et al., 2012; de Luis et al., 2015; Kitzman et al., 2016), which may be prescribed concomitantly. In this context, a very mild CR intervention (e.g. 10% deficit) or short intermittent fasting periods (> 14 hours and does not need to be daily) combined with exercise may have very positive effects and are likely to have higher compliance compered to more aggressive fasting or CR interventions.
Of course, severe and prolonged CR with no exercise (especially strength training) can induce lean mass loss that is highly undesirable if the goal is to improve health. Once again, and as with almost everything, the secret lies in the right dosage! In certain populations such as pregnant women (or women trying to conceive) and young growing individuals, prolonged CR interventions should be avoided as they may compromise development. However, I emphasize again that the most important thing is not to ingest “calories” but ingest “nutrients”! In older individuals with sarcopenia, CR should perhaps be avoided, although the most determining factors for reversing sarcopenia are strength training and adequate protein intake (which should be higher for older individuals (> 2g / kg bodyweight).
Regarding the specific case of intermittent fasting (note: the focus of this article is not to discuss the use of intermittent fasting as a fat loss and / or maintenance strategy or muscle mass gains in the sports context, but rather its potential for general health benefits) although few, the available randomized controlled trials in humans indicate that intermittent fasting do offer beneficial effects similar to those of constant CR and perhaps is easier to implement (Donati et al., 2008; Marzetti et al., 2009; Alirezaei et al., 2010; Arum et al.; 2014; Godar et al., 2015). Irregular fasting episodes (e.g. not eating breakfast once or twice a week on non-training days) can not only be a strategy easy to implement strategy as a mean to control total weekly calories ingested, and it also has positive hormonal effects is mediated by some of the mechanisms described above. “Hormesis” is defined as a mild stressor that is beneficial to health, stress resistance, growth and longevity. It results from exposure to an “adequate” dose of a stressor. CR or fasting (or exercise) is something that we are evolutionarily designed to tolerate, and which at the right dose gives us benefits and greater resilience. The occasional stress of not eating can be a “healthy discomfort”!
Until next time!
Nuno Correia
What mechanisms underlie the effects of calorie restriction or intermittent fasting on longevity and “age-related diseases”?
But after all what is “getting old” … and why …?
Aging has been characterized by several authors as a process of progressive deterioration of molecular, cellular and tissue structures and functions that is conditioned by genetic and environmental factors (Hu & Liu, 2014). This multifactorial and complex process resulting from this progressive loss of function makes the individual more vulnerable to disease and ultimately leads to death. The main determinants (resulting from genetic predisposition and environmental factors) that characterize the aging process at the cellular level have been identified as: free radical damage; mitochondrial dysfunction results in an accumulation of reactive oxygen species (ROS) and consequent oxidative stress; decrease and inefficiency of autophagy (an evolutionarily conserved process of recycling and “cellular waste removal” that is essential for cellular integrity, more details a bit ahead); changes in hormone-related signaling processes such as type 1 insulin-like growth factor (IGF-1), insulin and growth hormone; change in cholesterol and glucose metabolism; telomere shortening (Testa et al., 2014).
Now it seems that the aging process is indeed multifactorial. Probably the various aging theories (see part 1) are correct! In general, molecular processes are becoming more inefficient, slower and the system is progressively moving towards entropy. However, it seems that knowing the autophagy process (whose decline is associated with aging) may offer a “new” perspective on aging. Autophagy (or “self-digestion”) has been defined as an evolutionarily conserved (normal and important) catabolic process characterized by degradation in the lysosomes (cell organelle that functions as a “litter”) of damaged organelles, “defective” proteins and intracellular pathogens (Lavallard et al., 2012). Autophagy provides macromolecule degradation and recycling, not only providing new nutrients and energy during energy restriction (during calorie restriction or fasting), but also preventing the accumulation of cell metabolism by-products and protein aggregates in the cytoplasm. Therefore, autophagy is a protective and essential process for cellular homeostasis (Rubinsztein, Mariño & Kroemer, 2011) (note: rest assured that autophagy will “eat all the muscles” for a few hours without eating. That simply does not happen!). In fact, several authors have pointed deficient autophagic capacity as an important mediator of cellular senescence and consequent occurrence of “diseases or characteristics of old age” such as cardiovascular and neurodegenerative diseases; oxidative stress; weak immune system; chronic inflammation; osteoporosis; sarcopenia; diabetes; obesity; cancer (Pallauf & Rimbach, 2013; Pyo, Yoo & Jung, 2013). Specifically, reviews of mechanistic animal studies have indicated that loss of function in autophagy-related genes has resulted in intracellular accumulation of defective proteins and organelles and consequently in the acceleration of aging, while promoting autophagic activity increased life expectancy (Yen & Klionsky 2008)
(Note: Autophagy mechanisms have been in the mainstream news since 2016 with the Nobel Prize in Medicine 2016 awarded to the Japanese biologist Yoshinori Ohsumi. Their findings in autophagy mechanisms point in the direction that this cellular cleaning and recycling process is essential to prevent neurodegenerative and other diseases. This is the LINK for the news article).
Overall, and since the first rat studies by Dr. Clive McCay in 1935, calorie restriction has been extensively reviewed and recognized as a “potent” anti-aging strategy! Interventions in various types of animal species (from invertebrates to larger mammals such as primates) have shown that calorie restriction (without malnutrition) not only increases life expectancy (average and maximum), but delays the onset of so-called “age-related diseases ”(Martin, Mattson & Maudsley, 2006; Xiang & He, 2011; Lee & Min, 2013; Kitada & Koya, 2013b; Szafranski & Mekhail, 2014; Testa et al., 2014). The intermittent fasting regime (nothing more than another calorie restriction strategy as described in part 1 of this article) seems to offer the same kind of benefits (Martin, Mattson, & Maudsley, 2006; Robertson & Mitchell 2013).
Now the mechanisms by which calorie restriction or fasting induce health benefits appear to be (to a large extent) related to this antagonistic relationship between insulin signaling and autophagy. It is easy to understand, being autophagy a catabolic process (essential, normal and protective, I highlight that again) and the activation of insulin signaling pathways an anabolic process (equally important and essential in protein synthesis, insulin is not the “villain”), when one of these pathways is activated the other will be inhibited. Practically speaking, fasting activates the autophagy “machinery” and eating a meal (mainly containing protein and / or carbohydrates) activates the insulin signaling “machinery”. What seems to be essential is in fact that there are periods that allow the process of elimination and recycling provided by autophagy, and for this to happen it is necessary not to eat for a while. If there is no “room” for this process, due to constant food intake, it may lead to constant and “aberrant” insulin signaling state which may lead to many diseases that are usually associated with poor glucose and insulin metabolism which make the most of the so-called “age diseases”.
(Warning: the “less nerds“ should skip next paragraph)
Briefly, some of the mechanisms identified in animal studies that appear to underlie the health benefits induced by caloric restriction or intermittent fasting through regulation of autophagy and insulin pathway signaling are: 1) Inhibition of insulin / IGF-1signaling (due to the decrease in circulating amino acids and glucose) and its target pathways protein kinase B (PKB) / mammalian target of rapamycin (mTOR) ; 2) Activation of the sirtuin 1 pathway (SIRT1) due to the increase in NAD + / NADH ratio, which targets include activation of adenosine monophosphate protein kinase (AMPK), forkhead box O (FOXO) transcription factors, proliferator- activated gamma receptor-1-alpha coactivator (PGC-1α) (a mitochondrial biogenesis factor), and inhibition of the pro-inflammatory transcription factor NFkB; 3) Activation of the AMPK pathway due to the intracellular increase of the AMP / ATP ratio, which in turn induces up-regulation of FOXO and PGC-1α transcription factors and inhibition of the PKB / mTOR pathway. (Martin, Mattson & Maudsley, 2006; Han & Ren 2010; Rubinsztein, Mariño & Kroemer, 2011; Yen & Klionsky, 2008; Xiang & He, 2011; Pallauf, & Rimbach, 2013; Pyo, Yoo, & Jung, 2013; Hu & Liu, 2014; Szafranski & Mekhail, 2014; Amigo & Kowaltowski, 2014; Testa, G. et al., 2014; Madeo et al., 2015).
In humans, despite the smaller abundance of randomized controlled trials (for the reasons mentioned in Part 1 of this article), several reviews of intervention and observational studies (Yen & Klionsky, 2008; Marzetti, E. et al., 2009; Han & Ren 2010; Robertson & Mitchell, 2013; Testa et al., 2013; Madeo et al., 2015; Fan et al., 2016) indicate that the putative health benefits induced by calorie restriction or intermittent fasting are based on the same mechanisms related to insulin pathway signaling and regulation of autophagy. Some pointed benefits include: longer healthy longevity; better lipid profile; controlled blood pressure; optimization of diastolic and systolic function; better homeostatic control of insulin and glucose; better sensitivity to insulin and glucose; lower incidence of neurodegenerative diseases; lower adiposity; better mitochondrial biogenesis in the skeletal muscle; higher antioxidant capacity; lower levels of ROS and oxidative stress.
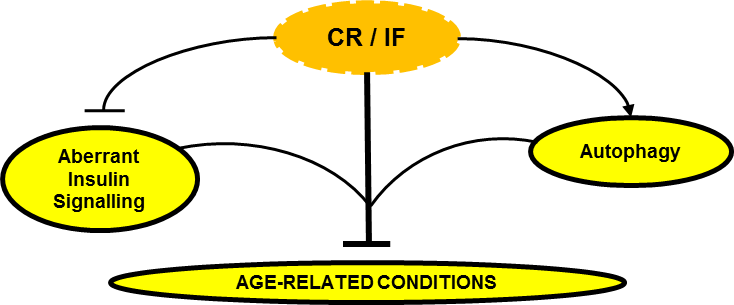
Can Calorie Restriction / Intermittent Fasting (CR / IF) alleviate age-related disease by regulating “aberrant” insulin signaling and autophagy?
In conclusion, the effect of caloric restriction or intermittent fasting on the regulation of insulin signaling and autophagy seems to emerge as a central regulatory axis that deserves attention (at least from me).
In the third part of this article I will then discuss what may be practical implications and applications of calorie restriction or fasting. Should we all do calorie restriction? Permanently? For how long? How much? What stage of life? Under what health conditions? Is the “intermittency” factor that delivers the best benefits?
Stay around!
Nuno Correia
References:
Amigo, I. & Kowaltowski, A.J., 2014. Dietary restriction in cerebral bioenergetics and redox state. Redox Biology, 2(1), pp.293–304.
Dröge W., 2009. Avoiding the First Cause of Death. New York, Bloomington. iUniverse, Inc.
Fan, J. et al., 2016. Autophagy as a Potential Target for Sarcopenia. Journal of Cellular Physiology, 231(7), pp.1450–1459. [Epub 2015 Dec 10].
Han, X. & Ren, J., 2010. Caloric restriction and heart function: is there a sensible link? Acta pharmacologica Sinica, 31(9), pp.1111–1117.
Hu, F. & Liu, F., 2014. Targeting tissue-specific metabolic signaling pathways in aging: the promise and limitations. Protein & cell, 5(1), pp.21–35.
Lavallard, V.J. et al., 2012. Autophagy, signaling and obesity. Pharmacological Research, 66(6), pp.513–525.
Lee, S.-H. & Min, K.-J., 2013. Caloric restriction and its mimetics. BMB reports, 46(4), pp.181–7.
Lee, S.-H. & Min, K.-J., 2013. Caloric restriction and its mimetics. BMB reports, 46(4), pp.181–7.
Lindeberg, S., 2010. Food and Western Disease: Health and Nutrition from an Evolutionary Perspective. Oxford, United Kingdom: Wiley-Blackwell.
Madeo, F. et al., 2015. Essential role for autophagy in life span extension. Journal of Clinical Investigation, 125(1), pp.85–93.
Martin, B., Mattson, M.P. & Maudsley, S., 2006. Caloric restriction and intermittent fasting: two potential diets for successful brain aging. Ageing research reviews, 5(3), pp.332–53.
Masoro.E. L., 2002. Caloric Restriction: A Key to Understanding and Modulating Aging. Texas, USA: ELSEVIER.
Pyo, J.O., Yoo, S.M. & Jung, Y.K., 2013. The interplay between autophagy and aging. Diabetes and Metabolism Journal, 37(5), pp.333–339.
Robertson, L.T. & Mitchell, J.R., 2013. Benefits of short-term dietary restriction in mammals. Experimental gerontology, 48(10), pp.1043–8.
Rubinsztein, D.C., Mariño, G. & Kroemer, G., 2011. Autophagy and aging. Cell, 146(5), pp.682–695.
Rubinsztein, D.C., Mariño, G. & Kroemer, G., 2011. Autophagy and aging. Cell, 146(5), pp.682–695.
Szafranski, K. & Mekhail, K., 2014. The fine line between lifespan extension and shortening in response to caloric restriction. Nucleus, 5(1), pp.56–65.
Testa, G. et al., 2014. Calorie restriction and dietary restriction mimetics: a strategy for improving healthy aging and longevity. Current pharmaceutical design, 20(18), pp.2950–77.
Xiang, L. & He, G., 2011. Caloric restriction and antiaging effects. Annals of Nutrition and Metabolism, 58(1), pp.42–48.
Yen, W.-L. & Klionsky, D.J., 2008. How to live long and prosper: autophagy, mitochondria, and aging. Physiology (Bethesda, Md.), 23(70), pp.248–262.
CAN CALORIE RESTRICTION OR INTERMITTENT FASTING HELP PREVENT “AGE-RELATED DISEASES” AND LIVE LONGER? – PART 1
Introduction
In this article I will look into the possibility of calorie restriction or intermittent fasting, which is just an alternative strategy to induce a caloric deficit, effective nutritional therapeutic strategies to prevent, alleviate or even eliminate some of the so-called “age-related diseases”, thus contributing for a better and longer life. It is important to note that most studies on the effects of calorie restriction or intermittent fasting (or intermittent calorie restriction) on life expectancy are mechanistic, and conducted in animal and / or in vitro models. It is understandable that there are fewer human intervention studies in this area. If we think about it, it is not easy to conduct studies with humans on calorie restriction to study its effects on life expectancy and the incidence of “age-related diseases”. Not only it is not easy to recruit people to voluntarily incur in a calorie restriction period, it is also not practical to study in humans (in a randomized controlled manner) the effects of calorie restriction or fasting on life expectancy, because they simply “live a long time”. ” In order to obtain timely results, it is essential to conduct studies on species with a shorter life expectancy. However, observational studies and some human intervention studies (discussed later) appear to confirm the same health benefits and molecular mechanisms as those observed in animals.
It should also be noted that, in the experimental context, caloric restriction is defined as “reduced food intake without malnutrition”. In other words, nutritional interventions imply a 10-40% reduction in daily caloric requirements in which only calories, and not nutrients, are restricted (in most controlled studies this is ensured with vitamin and mineral supplementation) (Kitada & Koya, 2013b; Robertson & Mitchell, 2013). This notion is important! Caloric deficit does not imply nutrient deficit and caloric surplus does not imply that nutrient requirements are met. Intermittent fasting will be no more than an alternative method of calorie restriction in which food intake is restricted for a certain period of time (usually 16 to 24 hours) followed by an unrestricted intake period and has been touted as producing beneficial health effects similar to more constant calorie restriction protocols (Martin, Mattson, & Maudsley, 2006; Robertson & Mitchell 2013).
Part 1
Should we accept being “sick” just because we get older?
It is recurrent to hear that the disease is something that “comes with the age package.” In fact, getting older is a drag! The general perception of a progressive decline of all our capabilities as we age is, unfortunately, not an illusion. There are several theories about aging. While certainly a very interesting topic, a detailed description of the various theories of aging is not the purpose of this article. These are some of mechanisms underlying the aging process which are generally pointed out as the main ones:
- The “Hayflick limit” (phenomenon discovered by Leonard Hayflick) determines that human cells have a replication limit number, after which they become senescent. Telomeres (i.e. a kind of protective “helmets” at the end of each chromosome) become progressively shorter with each cell division (Shay & Wright 2000). However, DNA methylation (an essential and repairing process consisting of the addition of methyl groups to DNA and which can be promoted by the abundance of dietary methyl donors for example) is said to be protective of telomere length and that way to postpone cell death and aging. For example, in animal models, hypomethylation of the enzyme telomerase reverse trancriptase has led to the preservation of leukocyte telomere length (Zhang et al. 2003; 2014). In this example, it is plausible to infer that delaying leukocyte senescence (through methylation and consequent telomere length conservation) may contribute to a stronger immune system and thus positively influence longevity.
- This theory suggests that unresolved chronic inflammation induces the human organism not to allocate resources for the functioning of other body functions (as they are permanently allocated to unresolved inflammation) and thus leading to early aging of various organs and tissues, and the early onset of “age-related diseases”.
- This theory, originally proposed by Dr. Denham Harman in 1956, is based on the premise that the aging process is mediated by free radical damage. Theoretically, by reducing free radical accumulation (e.g. reactive oxygen species) and at the same time increasing the antioxidant capacity of the organism (increasing glutathione, and antioxidant enzymes such as SOD and catalase), tissue damage can be prevented (by slowing down aging process) and to prevent the occurrence of “age-related diseases” and consequently contribute to increase functional longevity (Harman, 1988; 2006).
Very well, getting older is inevitable! We already know that. However, if we give it a little thought, all the mechanisms mentioned have an environmental root, that is, we can, to some extent, control them through decisions that we make every day. Namely decisions about what we eat and how we move. And this is good news! It is in fact in our hands to slow down the process of senescence and prevent the onset of the so-called ‘’age-related diseases’’. Note that if for us (Westernized world) it is statistically “normal” to grow old with diabetes, hypertension, cancer, dementia, sarcopenia, osteoporosis, cardiovascular disease, insulin resistance, obesity and chronic inflammation (because the population studied incurs in a lifestyle that leads to disease), in other contemporary (non-Westernized) populations such diseases are rare or even non-existent. In this context, I invite the reader to consult what I consider to be one of the best books I know about nutrition and lifestyle, and its relation to the incidence of so-called “Western” diseases: Food and Western Disease: Health and Nutrition from an Evolutionary Perspective by Staffan Lindeberg. In fact, if we want to aim at maximizing health and lifespan potential, we should not just look at what is ‘’normal’’ in a given population, because that may be a sick population. Rather, we should look for what is “biologically normal” for a human being! A species that is designed (evolutionarily) to deal with a range of environmental stimuli that include certain levels of physical activity, nutrition, sun exposure and sleep. And, although aging is a normal process, it should not be “biologically normal” to age with chronic disease.
In this context, the Okinawa Centenarian Study is also frequently cited. The Okinawan population has the highest ratio of (healthy) centenarians on the planet (50 / 100,000 vs 10-20 / 100,000 in the USA) and as such is of greatest interest to study the factors that potentiate this kind of longevity. One of the factors identified (in addition to an appreciable level of physical activity and social interaction) was the fact that populations over 70 eat about 11% of calories below (about 1785kcal / day, which is a very moderate level of calorie restriction) than would be recommended for maintaining body weight (according to the Harris-Benedict equation), however on a nutrient-rich diet (Wilcox et al., 2006).
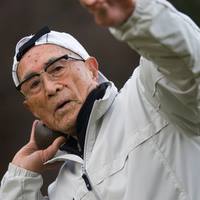
What we can do to live longer and better is one of my main interests. As I mentioned, our choices regarding the type of exercise, food we eat and other lifestyle factors can affect how long we live and, perhaps most importantly, how healthy and functional we live. In Part 2 of this article I will discuss some mechanisms by which nutritional interventions such as calorie restriction or intermittent fasting can lead to health benefits. And in Part 3 I will address the possible implications and practical applications of the practice of calorie restriction or fasting, as well as which populations can benefit most from these nutritional strategies and whoshould avoid them.
Stay around!
Nuno Correia
References
Dröge W., 2009. Avoiding the First Cause of Death. New York, Bloomington. iUniverse, Inc.
Harman D., 1988. Free radicals in aging. Mol Cell Biochem. Dec; 84(2), pp.155-161.
Harman D., 2006. Free radical theory of aging: an update: increasing the functional life span. Ann N Y Acad Sci. May;1067, pp.10-21.
Kitada, M. & Koya, D., 2013b. SIRT1 in Type 2 Diabetes: Mechanisms and Therapeutic Potential. Diabetes & metabolism journal, 37(5), pp.315–25.
Lindeberg, S., 2010. Food and Western Disease: Health and Nutrition from an Evolutionary Perspective. Oxford, United Kingdom: Wiley-Blackwell.
Martin, B., Mattson, M.P. & Maudsley, S., 2006. Caloric restriction and intermittent fasting: two potential diets for successful brain aging. Ageing research reviews, 5(3), pp.332–53.
Masoro.E. L., 2002. Caloric Restriction: A Key to Understanding and Modulating Aging. Texas, USA: ELSEVIER.
Robertson, L.T. & Mitchell, J.R., 2013. Benefits of short-term dietary restriction in mammals. Experimental gerontology, 48(10), pp.1043–8.
Shay J.W., Wright W.E. 2000. Hayflick, his limit, and cellular ageing. Nat Rev Mol Cell Biol. Oct;1(1), pp.72-76.
Zhang D. et al., 2013. Homocysteine-related hTERT DNA demethylation contributes to shortened leukocyte telomere length in atherosclerosis. Atherosclerosis. Nov; 231(1), pp.173-179.
Zhang D.H., Wen X.M., Zhang L. & Cui W., 2014. DNA methylation of human telomerase reverse transcriptase associated with leukocyte telomere length shortening in hyperhomocysteinemia-type hypertension in humans and in a rat model. Circ J. 78(8), pp.1915-1923.
Wilcox D.C. et al., 2006. Caloric restriction and human longevity: what can we learn from the Okinawans? Biogerontology 7, pp.173–177.